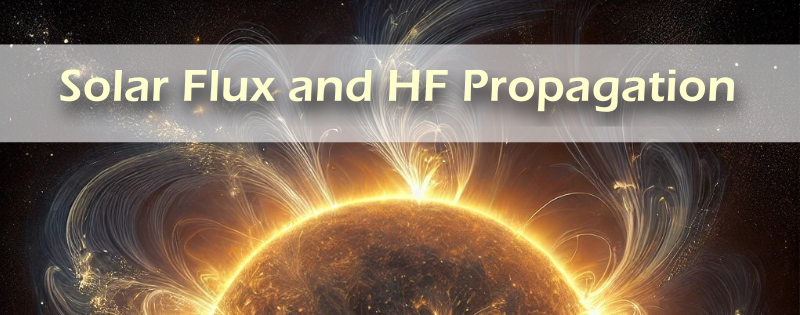
HF Propagation: How Solar Flux and the Ionosphere Shape Radio Communication
HF propagation—the ability of high-frequency radio waves to travel long distances—relies on the complex interaction between solar activity and the Earth’s ionosphere. At the core of this process is solar flux, which drives ionization of the ionosphere and determines how effectively radio signals are reflected back to Earth.
In this post, I will explore a few topics:
- The basics of HF propagation and the role of solar flux.
- The physics behind how the ionosphere interacts with radio waves.
- Practical tips for optimizing HF communications based on solar and other factors.
What is HF Propagation?
HF propagation refers to the way radio signals in the HF band (3 to 30 MHz) travel over long distances by interacting with the Earth’s ionosphere. Unlike lower frequency signals that hug the Earth’s surface, or higher frequency signals that travel in straight lines—often shooting right through the ionosphere and into outer space—HF waves can “bounce” between the ionosphere and the ground or within stratified portions of the ionosphere itself. This unique characteristic allows these signals to travel far beyond the horizon, enabling global communications.
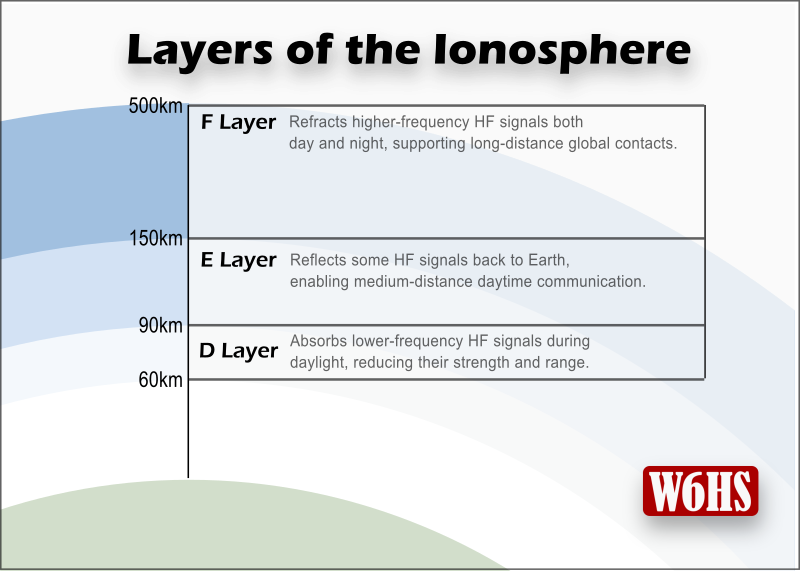
What is Solar Flux?
In physics, flux refers to the amount of energy passing through a given area in a specific amount of time. For solar flux, this energy is in the form of electromagnetic radiation emitted by the Sun.
Solar flux is measured in units of watts per square meter per hertz (W/m²/Hz). It quantifies how much solar radio energy reaches a one-square-meter area of the Earth’s surface, at our given distance from the Sun, for each unit of radio frequency or oscillation. It sounds complex, but it’s really straightforward.
In practical terms for radio communications, flux simply indicates the Sun’s level of activity. A higher flux value means more energy is being emitted by the Sun, enhancing ionization of the atmosphere, thus improving reflectivity of the ionosphere at higher frequencies. Lower flux numbers indicate less energy and thus less reflective/conductive plasma for a radio signal to interact with.
Solar Flux and the F10.7 Index
Solar flux is measured at a wavelength of 10.7cm (2.8GHz). This measurement, known as the F10.7 Index, serves as an indicator for solar emissions across a wide range of frequencies, including UV and X-Ray. It’s these high-energy emissions that ionize atmospheric gases, creating the ionosphere. The appearance of sunspots is a direct indicator of the Sun’s magnetic activity. Higher sunspot numbers correlate with stronger emissions at 10.7cm, making the F10.7 index a reliable proxy for solar activity. Since the majority of 10.7cm radiation originates from the corona and chromosphere of the Sun, primarily near regions of active sunspots.
Solar Radiation, Plasma, and the Upper Atmosphere

The ionosphere is an upper atmosphere layer that becomes reflective to radio waves when solar activity, particularly solar flux, excites atomic and molecular components in this layer, causing them to to shed electrons. It’s these free electrons that make plasma what it is: An electrically-conductive supercharged gas-like substance that exhibits properties of both matter and energy. This plasma is the basis for HF propagation. It is the electrically-active component of the atmosphere that puts the magic in ham radio.
The Physics of HF Propagation
HF propagation is made possible by the interaction between radio waves and the ionosphere’s plasma, which consists of free electrons with negative charge and positive ions left behind from the ionization process. The electric field of the HF radio signal interacts with the free electrons in the ionosphere, causing them to oscillate. These oscillations then generate secondary electromagnetic waves, either cancelling or reinforcing the original signal.
As radio waves travel through the ionosphere, they encounter regions of varying electron density resulting in phase shifts, audible distortion of received signal, and bending or refracting of the original signal. It’s these sometimes oscillating distortions that make tuning a distance station so much fun.
Counter to the propagation-promoting electrical fields of the upper layers, there is absorption to contend with in the D-layer, where radio signals cause free electrons to oscillate in time with the signal as it passes through. As this happens, these free electrons collide over and over again with oxygen, nitrogen, and neutral molecules in the lower, denser part of the atmosphere. These collisions generate friction, robbing the otherwise liberated electrons of their kinetic energy, which in turn robs energy from the original radio signal, resulting in sometimes notable attenuation at certain frequencies.
Factors Influencing HF Propagation
There are several factors that can influence HF propagation aside from the solar flux and free electrons we just learned about. Let’s take a look at some of these other fascinating factors that enhance our understanding of HF propagation:
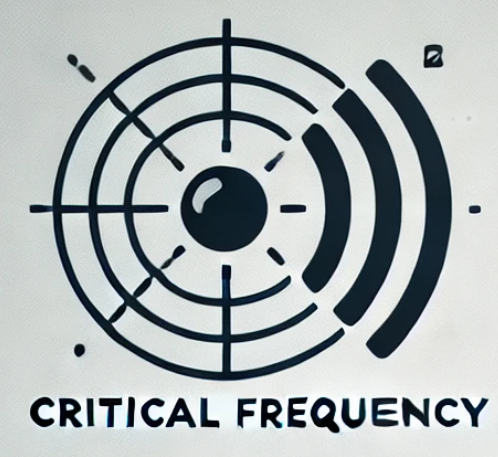
Critical Frequency
The critical frequency is the highest frequency at which a radio wave can travel directly upward and still be reflected back to Earth by the ionosphere, rather than passing through it into space. It depends on the density of free electrons in the ionosphere, which varies with solar flux and atmospheric conditions.
The critical frequency is an important concept in radio communications, as it helps determine which frequencies can be used for reliable short- and long-distance propagation. Frequencies below the critical frequency will reflect back, while those above it may pass through unless transmitted at an angle.
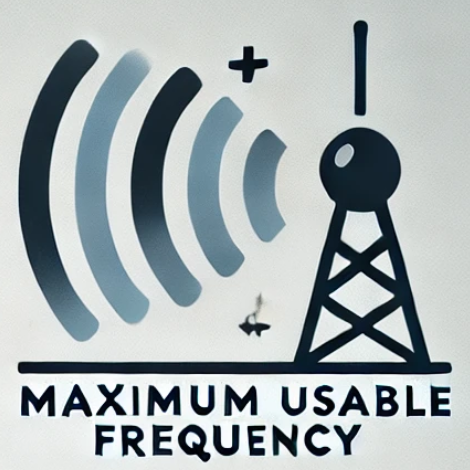
Maximum Usable Frequency (MUF)
The Maximum Usable Frequency (MUF) is the highest frequency that a radio signal can use to successfully reflect off the ionosphere and return to Earth, rather than passing through it into space. It depends on factors such as solar activity, time of day, and the angle at which the signal is transmitted.
Higher solar activity typically increases the MUF, making higher frequency bands usable for long-distance communication. However, if a frequency exceeds the MUF, the signal will not reflect and will instead be lost to space. Understanding and predicting the MUF is crucial for ham radio operators and other communicators to optimize their signal’s range and clarity.
While critical frequency and maximum usable frequency may sound like two descriptions of the same thing, the important distinction is that MUF describes reflectivity of signals at oblique angles, while CF describes signals sent straight up and is used as a scientific measurement tool. In other words, MUF describes ionospheric reflectivity that’s actually beneficial to ham radio operations.
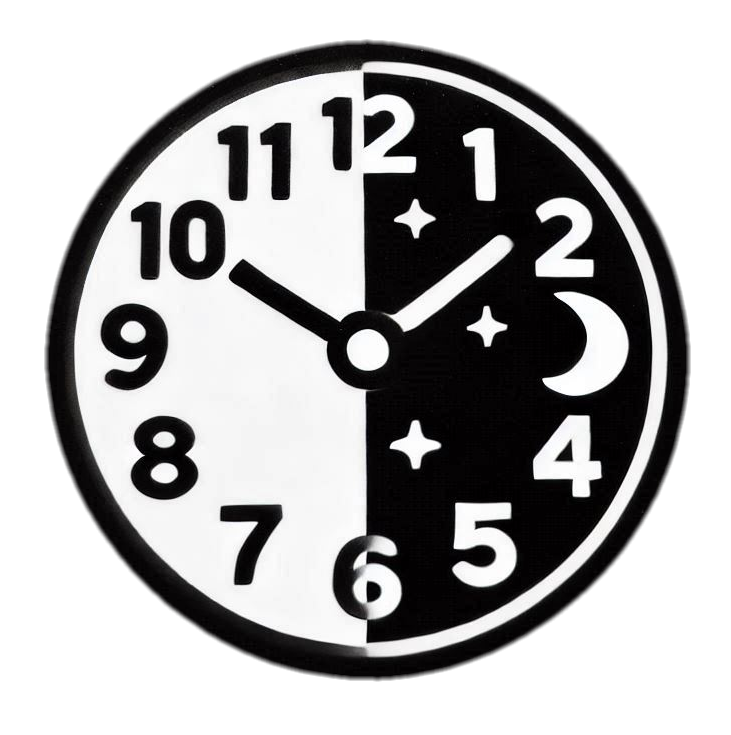
Time of Day
HF bands work very differently from day to night. The 10-meter band, for example, has a high enough frequency that it manages to get through the D-layer without attenuating out of existence, but rarely works at night because the 28MHz band requires more energetic E and F layers and these layers have only residual ionization after dark. As the D-layer cools down at night, lower frequencies are allowed to pass through.
- Daytime: Solar radiation strengthens ionization, improving higher HF band propagation.
- Nighttime: The D-layer disappears, reducing absorption, but lower ionization in the F-layer lowers the MUF. Lower bands become more effective.
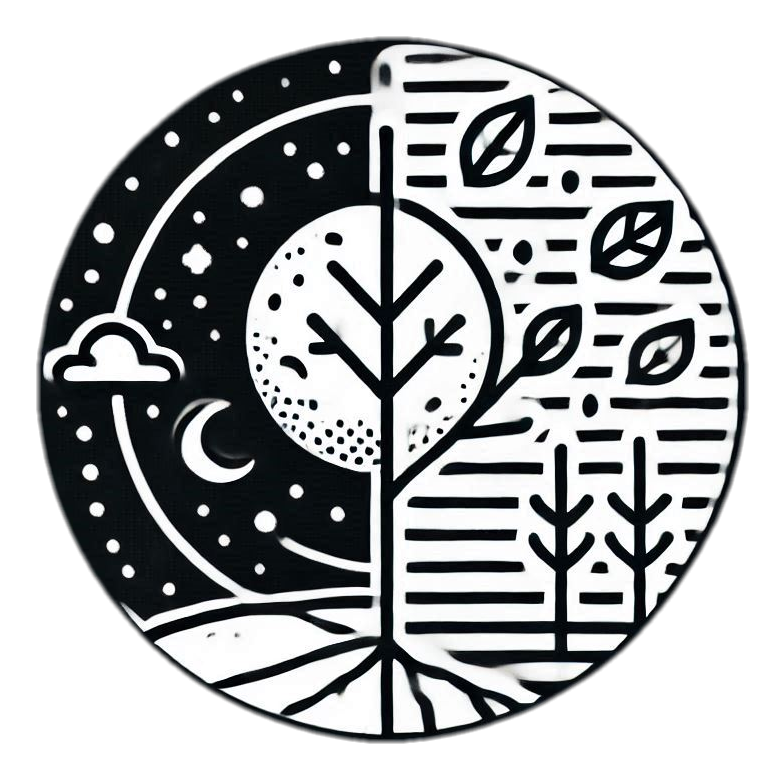
Seasonal Effects
Seasonal changes play a significant role in HF radio propagation as well. In summer, longer daylight hours and increased solar radiation enhance ionization in all three layers, making higher HF frequencies more effective for long-distance communication. However, the stronger D-layer absorption during the day can dampen lower HF frequencies like 80 and 40 meters.
Conversely, during the winter months, shorter days and weaker solar radiation reduce ionization, lowering the maximum usable frequency. This shift makes the lower HF bands better suited for nighttime communication, as there is less D-layer absorption and less interference from solar activity.

Sporadic-E Propagation
In addition to seasonal ionization trends, specific phenomena like Sporadic-E propagation show strong seasonal variations. Sporadic-E, where highly ionized patches in the E-layer reflect HF signals, is most prevalent in late spring and early summer. This makes bands like 10 and 6 meters highly active during these periods, allowing short-to-medium distance communication that might otherwise be impossible.
Winter, on the other hand, often brings quieter ionospheric conditions with less Sporadic-E activity but increased stability on lower bands for long-haul propagation. Understanding these seasonal dynamics helps radio operators optimize their frequency choices and timing for reliable communication year-round.
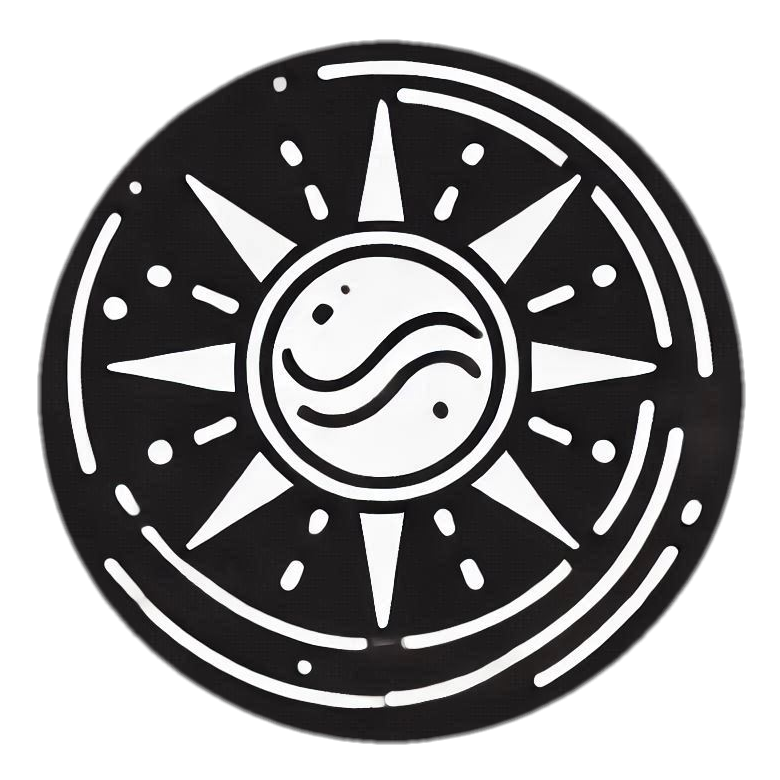
Solar Cycle
The solar cycle, an 11-year cycle of varying solar activity, has a significant impact on HF propagation. The peak of this cycle, known as solar maximum, is happening right now. During solar maximum, the Sun emits increased ultraviolet (UV) and X-ray radiation, which greatly enhances ionization in the Earth’s ionosphere, particularly in the F-layer, resulting in better propagation at higher frequencies.
At solar maximum, the critical frequency of the ionosphere increases, supporting reliable long distance propagation at frequencies that might otherwise escape into space. Operators can take advantage of these conditions to make contacts across continents and oceans with relatively low power.
Conversely, during the solar minimum, when solar activity is generally at its lowest, the reduced radiation leads to lower ionization. This causes the critical frequency to drop, limiting the use of higher HF bands like 10 meters, which often remain closed for long-distance propagation.
Instead, lower HF bands such as 40, 80, and 160 meters become more reliable, especially at night when the D-layer absorption diminishes. While solar minimum conditions limit the performance of higher frequencies, they can offer improved propagation on low bands, with less interference and atmospheric noise. Understanding where we are in the solar cycle allows radio operators to adjust their band choices and operating strategies to maximize communication opportunities.

Space Weather: Extreme Solar Events
Coronal Mass Ejections (CMEs) and solar flares are intense solar events that can severely disrupt HF communications. Solar flares are sudden bursts of energy on the Sun’s surface that emit powerful X-rays and ultraviolet (UV) radiation, which travel to Earth at the speed of light.
When these high-energy emissions reach the Earth’s atmosphere, they cause rapid and intense ionization in the D-layer of the ionosphere. This increase in ionization leads to radio wave absorption, particularly for lower HF frequencies (below 10 MHz). Such events, known as shortwave fadeouts, occur on the sunlit side of the Earth and can completely block HF signals for minutes to hours, depending on the flare’s intensity.
On the other hand, CMEs involve massive clouds of charged particles ejected from the Sun, traveling through space at speeds of up to millions of kilometers per hour. When a CME reaches Earth, it interacts with the planet’s magnetic field, causing geomagnetic storms.
These storms disturb the ionosphere, creating irregular and unpredictable ionization patterns that can severely affect HF signal propagation. Operators may experience signal fading, sudden changes in propagation paths, and increased noise levels.
Polar regions are particularly vulnerable, as charged particles entering the atmosphere tend to do so at the poles, exacerbating disruptions in these areas. Together, solar flares and CMEs can create challenging conditions for HF operators, sometimes rendering communication unreliable or completely unavailable for extended periods.
Practical Tips for HF Radio Operators
To make the most of HF propagation, ham radio operators must learn to adapt their strategies based on time, frequency, and solar conditions. First, pay attention to the time of day when planning contacts. Higher frequency bands, such as 15, 12, and 10 meters, work best during the day when the ionosphere is more heavily ionized. Lower frequency bands like 40, 80, and 160 meters are better suited for nighttime, as the D-layer dissipates, reducing signal absorption. Use propagation tools like VOACAP or real-time ionospheric maps to predict which bands are likely to be open for long-distance contacts at any given time.
Stay aware of solar activity and seasonal changes to further optimize your operating times and band choices. During solar maximum, take advantage of the higher ionospheric critical frequencies to work the 10- and 15-meter bands, which can offer excellent global coverage with minimal power. Keep an eye on space weather reports for solar flares and geomagnetic storms, as these events can disrupt HF communication. Additionally, experiment with your antenna setup—higher elevation and directional antennas like Yagis can improve signal strength and directionality, while simple dipoles can still perform well on lower bands. By combining knowledge of propagation conditions with strategic band selection and equipment adjustments, you’ll maximize your chances of successful contacts across the globe.
Final thoughts on Solar Flux and HF Propagation
Solar flux is the driving force behind HF propagation, determining how well HF radio waves interact with the ionosphere. By understanding how ionospheric layers behave under different solar conditions, radio operators can make better decisions about frequency selection and timing.